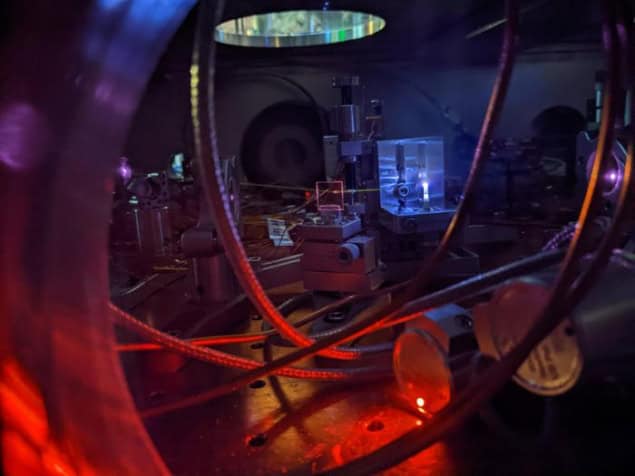
Could a new type of clock potentially be more accurate than today’s best optical atomic clocks? Such a device is now nearing reality, thanks to new work by researchers at JILA and their collaborators who have successfully built all the elements necessary for a fully functioning nuclear clock. The clock might not only outperform the best time-keepers today, it could also revolutionize fundamental physics studies.
Today’s most accurate clocks rely on optically trapped ensembles of atoms or ions, such as strontium or ytterbium. They measure time by locking laser light into resonance with the frequencies of specific electronic transitions. The oscillations of the laser then behave like (very high-frequency) pendulum swings. Such clocks can be stable to within one part in 1020, which means after nearly 14 billion years (or the age of the universe), they will be out by just 10 ms.
As well as accurately keeping time, atomic clocks can be used to study fundamental physics phenomena. Nuclear clocks should be even more accurate than their atomic counterparts since they work by probing nuclear energy levels rather than electronic energy levels. They are also less sensitive to external electromagnetic fluctuations that could affect clock accuracy.
Detecting tiny temporal variations
A nucleus measures between 10-14 and 10-15 m across, while an atom is 10-10 m. Shifts between nuclear energy levels are thus higher in energy and would be resonant with a higher-frequency laser. This translates into more wave cycles per second — and can be thought of as a greater number of pendulum swings per second.
Such a nuclear transition probes fundamental particles and interactions differently to existing atomic clocks. Comparing a nuclear clock with a precise atomic clock could therefore help to unearth new discoveries related to very tiny temporal variations, such as those in the values of the fundamental constants of nature. Any detected changes would point to physics beyond the Standard Model.
The problem is that the high-frequency lasers needed to excite the nuclear transitions in most elements are not easy to come by. To excite nuclear transitions, most atomic nuclei need to be hit by high-energy X-rays. In the late 1970s, however, physicists identified thorium-229 as having the smallest energy gap of all atoms and found that it could thus be excited by lower-energy, ultraviolet light. In 2003, Ekkehard Peik and Christian Tamm at the Physikalisch-Technische Bundesanstalt (Germany’s National Metrology Institute), proposed that this transition could be used to make a nuclear clock. But, it was only in 2016 that this transition was directly observed for the first time.
In the new study, an international team led by Jun Ye at JILA, a joint institute of NIST and the University of Colorado Boulder, have fabricated all of the components needed to create a nuclear clock made from thorium-229. These are: a coherent laser for resolving different nuclear states; a “high concentration” thorium-229 sample embedded in a solid-state calcium fluoride host crystal; and a “frequency comb” referenced to an established atomic standard for precisely measuring the frequency of these transitions.
A frequency comb is a special type of laser that acts like a measuring stick for light. It works using laser light that comprises up to 106 equidistant, phase-stable frequencies (which look like the teeth of a comb) to measure other unknown frequencies with high precision and absolute traceability when compared with a radiofrequency standard. The researchers used a frequency comb operating in the infrared part of the spectrum, which they upconverted (through a cavity-enhanced high harmonic generation process) to produce a vacuum-ultraviolet frequency comb whose frequency is linked to the infrared comb. They then used one line in the comb laser to drive the thorium nuclear transition.
Comparisons for fundamental physics studies
And that is not all: the team also succeeded in directly comparing the ultraviolet frequency to the optical frequency employed in one of today’s best atomic clocks made from strontium-87. This last feat will be the starting point for future nuclear–atomic clock comparisons for fundamental physics studies. “For example, we’ll be able to precisely test if some fundamental constants (like the fine structure alpha) are constant or slowly varying over time,” says Chuankun Zhang, a graduate student in Ye’s group.
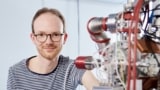
Nuclear clocks: why an experiment at CERN brings them closer to reality
Looking forward, the researchers say that they eventually hope to use their technology to make portable solid-state nuclear clocks that can be deployed outside the laboratory for practical applications. They also want to investigate how the clock transitions shift depending on temperature and different crystal environments.
“We also plan to develop faster readout schemes of the excited nuclear states for actual clock operation,” Zhang tells Physics World.
The study is detailed in Nature.