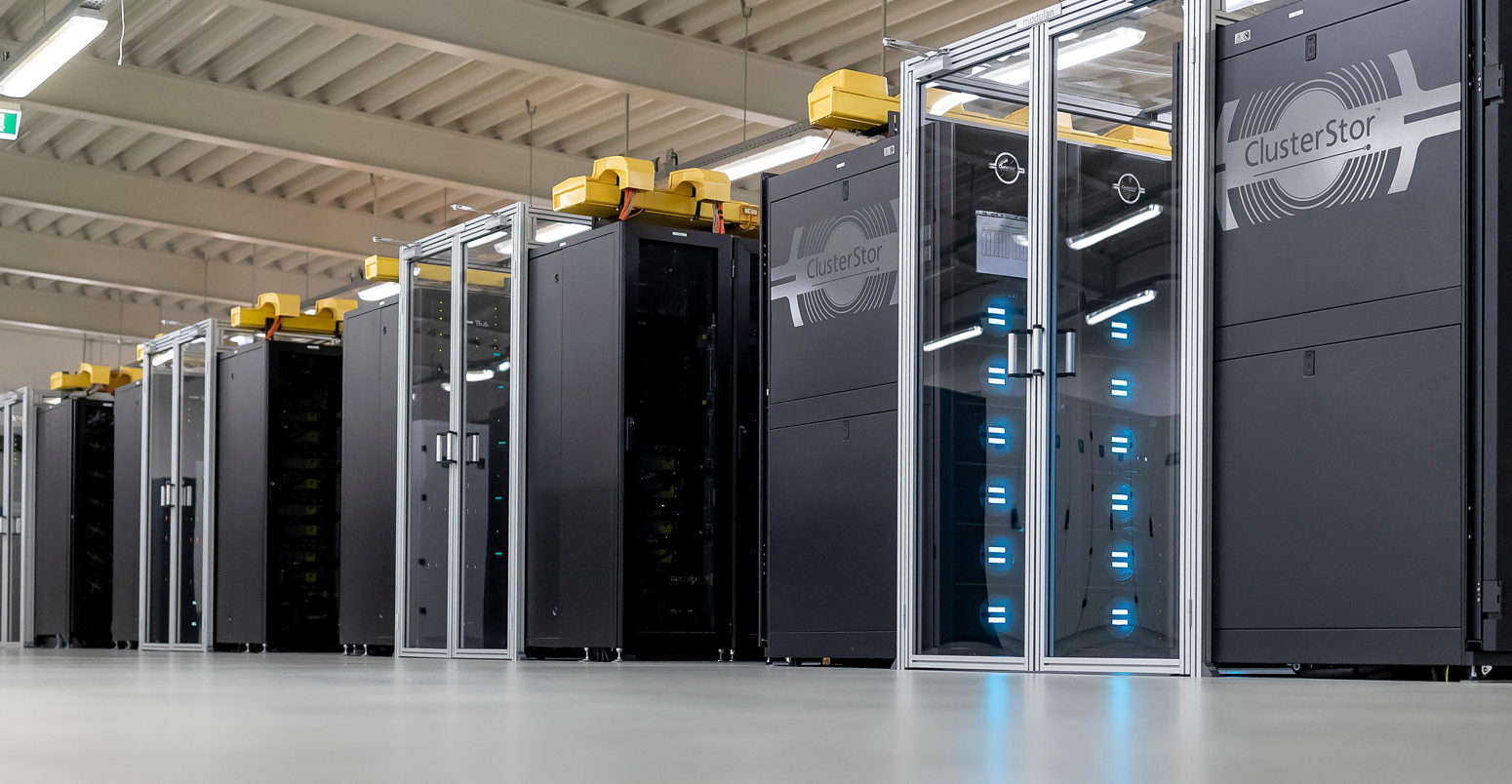
CMIP6: the next generation of climate models explained

Zeke Hausfather
12.02.19
Zeke Hausfather
02.12.2019 | 8:00amClimate models are one of the primary means for scientists to understand how the climate has changed in the past and may change in the future. These models simulate the physics, chemistry and biology of the atmosphere, land and oceans in great detail, and require some of the largest supercomputers in the world to generate their climate projections.
Climate models are constantly being updated, as different modelling groups around the world incorporate higher spatial resolution, new physical processes and biogeochemical cycles. These modelling groups coordinate their updates around the schedule of the Intergovernmental Panel on Climate Change (IPCC) assessment reports, releasing a set of model results – known as “runs” – in the lead-up to each one.
These coordinated efforts are part of the Coupled Model Intercomparison Projects (CMIP). The 2013 IPCC fifth assessment report (AR5) featured climate models from CMIP5, while the upcoming 2021 IPCC sixth assessment report (AR6) will feature new state-of-the-art CMIP6 models.
CMIP6 will consist of the “runs” from around 100 distinct climate models being produced across 49 different modelling groups. The effort is already a year behind schedule, and it appears increasingly unlikely that all the CMIP6 models will be available in time for inclusion in the AR6.
Enough data is now available for Carbon Brief to produce an assessment of how things have changed since CMIP5. These models are running a number of new and updated emission pathways that explore a much wider range of possible future outcomes than were included in CMIP5.
While the results from only around 40 CMIP6 models have been published so far, it is already evident that a number of them have a notably higher climate sensitivity than models in CMIP5. This higher sensitivity contributes to projections of greater warming this century – around 0.4C warmer than similar scenarios run in CMIP5 – though these warming projections may change as more models become available. Researchers are still working to assess why sensitivity values appear higher in the latest generation of models.
In this explainer, Carbon Brief provides an overview of the future emissions scenarios that are being used in CMIP6. This includes an examination of the climate sensitivity, past and future warming in CMIP6 models released so far, and a look at how they compare with the prior generation of models in CMIP5. Finally, this article will summarise the different experiments that climate models are undertaking in CMIP6.
Update 3 November 2020: With many additional CMIP6 runs now available from the ScenarioMIP project, this post has been updated to reflect the results. The original version of this article included only 14 CMIP6 model runs; this update now includes 42 historical runs and up to 35 runs in some future emission scenarios. All of the figures in both the “CMIP6 historical simulations” and “Future warming in CMIP6” sections have been updated to reflect the latest results. The original charts can be viewed at this archived version of the article.
Update 17 June 2020: This article has been updated to include more model sensitivity estimates, as well as a new section about the latest studies looking at the reliability of higher sensitivity models.
Future emission scenarios
CMIP6 represents a substantial expansion over CMIP5, in terms of the number of modelling groups participating, the number of future scenarios examined and the number of different experiments conducted.
The goal of CMIP is to generate a set of standard simulations that each model will run. This allows results to be directly comparable across different models, to see where models agree and disagree on future changes. One of the main sets of simulations run by models are future climate scenarios, where models are given a common set of future concentrations of greenhouse gases, aerosols and other climate forcings to project what might happen in the future.
In the lead up to the IPCC AR6, the energy modelling community has developed a new set of emissions scenarios driven by different socioeconomic assumptions, These are the “Shared Socioeconomic Pathways” (SSPs). A number of these SSP scenarios have been selected to drive climate models for CMIP6.
Specifically, a set of scenarios were chosen to provide a range of distinct end-of-century climate change outcomes. The IPCC AR5 featured four Representative Concentration Pathways (RCPs) that examined different possible future greenhouse gas emissions. These scenarios – RCP2.6, RCP4.5, RCP6.0, and RCP8.5 – have new versions in CMIP6. These updated scenarios are called SSP1-2.6, SSP2-4.5, SSP4-6.0, and SSP5-8.5, each of which result in similar 2100 radiative forcing levels as their predecessor in AR5.
A number of new scenarios are also being used for CMIP6 in order to give a wider selection of futures for scientists to simulate. These scenarios are included in the chart below, which shows the annual CO2 emissions assumed under each scenario out to 2100. The new scenarios include SSP1-1.9 (purple line), SSP4-3.4 (blue solid), SSP5-3.4OS (blue dashed) and SSP3-7.0 (orange).
One major improvement to CMIP6 scenarios is a better exploration of possible baseline “no climate policy” outcomes. The prior generation of climate models featured in CMIP5 only included one very high baseline scenario (RCP8.5) and one relatively little-mitigation scenario consistent with baseline outcomes (RCP6.0). Much of the subsequent literature relied on RCP8.5 as the only no-policy baseline, often referring to it as “business as usual” despite it being somewhat of a worst-case among possible no-policy outcomes.
CMIP6 has added a new scenario – SSP3-7.0 – which lies right in the middle of the range of baseline outcomes produced by energy system models. Now modellers can examine worst case (SSP5-8.5), middle of the road (SSP3-7.0) and more optimistic (SSP4-6.0) outcomes when modelling how the world might warm in a world that fails to enact any climate policies.
SSP4-3.4 is another new scenario that tries to explore the space between scenarios that generally limit warming to below 2C (RCP2.6 / SSP1-2.6) and around 3C (RCP4.5 / SSP2-4.5) by 2100. It will help scientists better assess the impacts of warming if societies rapidly reduce emissions, but fail to mitigate fast enough to limit warming to below 2C.
SSP5-3.4OS is an overshoot scenario (OS) where emissions follow a worst-case SSP5-8.5 pathway until 2040, after which they decline extremely rapidly with a lot of late-century use of negative emissions.
Finally, SSP1-1.9 is a scenario intended to limit warming to below 1.5C by 2100 above pre-industrial levels. It was added in the aftermath of the Paris Agreement when countries agreed to pursue efforts to limit the temperature increase to 1.5C. The energy models and simple climate models developed to limit warming to 1.5C played a big role in the special report on 1.5C that the IPCC published in 2018. These new CMIP6 scenarios will now allow full climate models to explore climate changes and impacts at around 1.5C warming.
CMIP6 features new scenarios that result in 2100 forcing similar to the CMIP5 RCP scenarios. However, even though their end-of-century forcing is the same, the emissions pathways and mix of CO2 and non-CO2 emissions are different. The figure below compares the CO2 emissions in the old RCP scenarios (dashed lines) and their new SSP counterparts (solid lines).
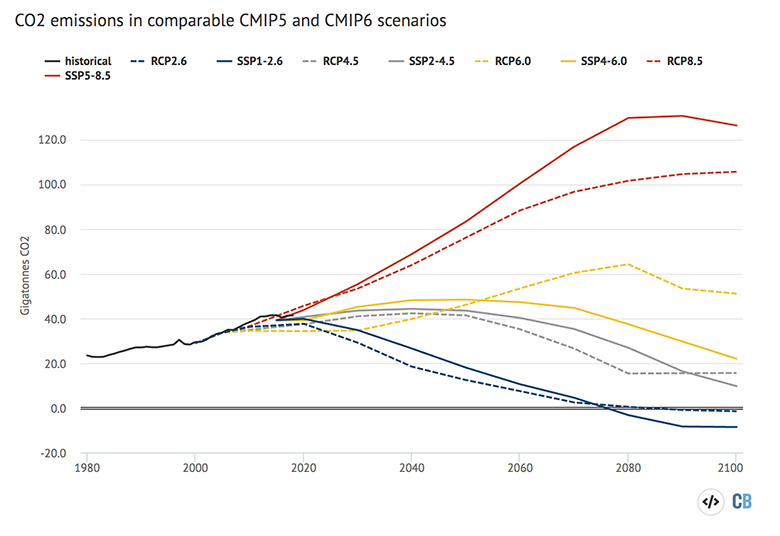
There are several reasons for these differences. The new SSP scenarios start in 2014, while the old RCPs started in 2007. The low-end SSP1-2.6 scenario shows a more gradual decline in emissions than RCP2.6 and a higher starting point, reflecting in part that 2007-2014 emissions have been notably higher than expected in the original RCP2.6 scenario. It employs substantially more late-century negative emissions to make up for the higher starting point and slower decline.
SSP2-4.5 also shows a higher starting point and slightly slower decline than RCP4.5, though larger non-CO2 emission declines in SSP2-4.5 also play a role. SSP4-6.0 is quite different from RCP6.0, with CO2 emissions peaking and declining after 2050 compared to 2080. This faster mitigation offsets higher near-term emissions, though emissions of greenhouse gases other than CO2 also play a role. Finally, SSP5-8.5 has substantially higher CO2 emissions than RCP8.5, with correspondingly larger cuts in non-CO2 emissions.
Many higher sensitivity models
Equilibrium climate sensitivity (ECS) is the expected long-term warming after a doubling of atmospheric CO2 concentrations. It is one of the most important indicators of how severe the impacts of future warming will be. “Sensitivity” is something that emerges from the physical and biogeochemical simulations within climate models; it is not something that is explicitly set by modellers.
CMIP6 models available to-date have tended to show notably higher climate sensitivity than CMIP5 models. While only 40 CMIP6 models currently have the runs needed to calculate ECS, around a third of those have an ECS higher than the upper end of the likely range – 1.5C to 4.5C – provided in the IPCC AR5. A quarter have a higher sensitivity than any of the models featured in CMIP5. These include models from a number of very prominent modeling groups – like the Community Earth System Model 2 (CESM2) and the Met Office HadGEM3 model. The transient climate response – a measure of shorter-term warming associated with increasing CO2 – is also notably higher in many CMIP6 models.
The figure below shows the IPCC AR5 likely ECS range (black bar), the range and individual values for CMIP5 models (grey bar; black dots), as well as the range and values of CMIP6 models available to-date (blue bar; black dots).
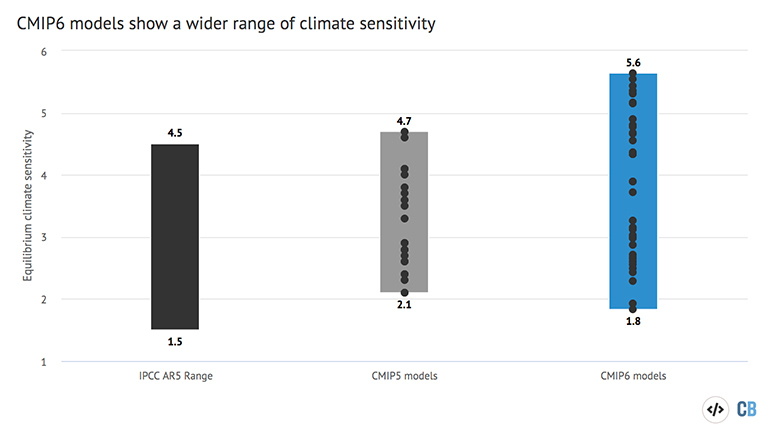
Researchers are currently looking into what is driving these high ECS values. In a number of models the increase in ECS appears to be due to their improved representation of clouds and aerosols; for example, how models treat supercooled clouds (below freezing but still liquid) in the Southern oceans can make a big difference in resulting sensitivity.
However, despite making the models more realistic, it is not yet clear whether these improvements are translating into more accurate estimates of ECS. For example, a number of climate scientists have expressed scepticism of the high-end values, arguing that they are inconsistent with evidence from palaeoclimate records and other lines of evidence.
It remains to be seen how the IPCC AR6 will reconcile the high ECS from some models with other sources of evidence, and if they will update the “likely” sensitivity range.
The ECS values for each of the 40 CMIP6 models available so far are shown in the figure below. Fourteen of these, highlighted in yellow, have an ECS above 4.5C, and eleven of these have sensitivities above that of the highest model in CMIP5 (e.g. 4.7C). Twenty six models have ECS values consistent with the AR5 range, with the lowest ECS model in CMIP6 (1.8C) similar to the lowest in CMIP5 (2.1C).
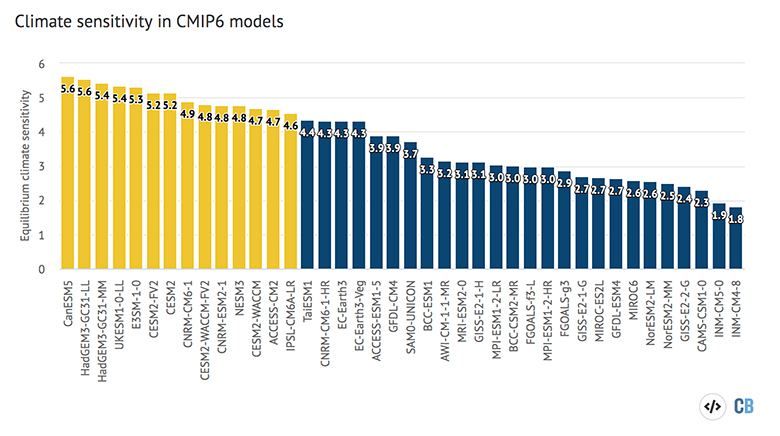
AR5’s “likely” ECS range of 1.5C-4.5C means that there is a 66% chance that the true value falls in that range. Therefore, around 33% of values can be expected to fall outside this range – and there are good reasons to expect that values higher than 4.5C are more likely than values below 1.5C. For example, evidence from palaeoclimate data, models, and observations all suggests that an ECS of under 1.5C is unlikely, while they do not as easily rule out an ECS of above 4.5C.
Having a diverse range of ECS values in CMIP6 is not necessarily a bad thing, as it indicates that modellers are not making choices to make their results similar to those of other modeling groups (e.g. fitting in with the herd). However, the fact that a number of models available so far have a very high ECS means either future warming may be worse than we thought or a number of prominent climate models may be getting climate sensitivity wrong, a question that many climate scientists are focusing on resolving.

Latest studies
Recently, a number of studies have tried to address the reliability of higher sensitivity models. Two studies – one by Femke Nijsse and colleagues at the University of Exeter and another by Dr Katarzyna Tokarska and colleagues at ETH Zurich – point out that the highest sensitivity models do a poor job of reproducing historical temperatures, either showing no 20th-century warming and too-rapid recent warming, or too much overall warming. They suggest that historical temperatures can serve as an emergent constraint to help rule out the new very-high sensitivity estimates.
On the other hand, some researchers have argued that natural variability has helped produce a pattern of warming in recent years that leads to lower global temperatures than would otherwise have occurred, making it challenging to use observed warming to constrain future projections.
A recent study by Dr Keith Williams and colleagues at the UK Met Office suggested that the latest Met Office model – which has a sensitivity of 5.5C per doubling CO2 – performs much better in producing short-term forecasts than variants of the model with a lower climate sensitivity as it has greater amounts of cloud liquid water at cold temperatures.
However, as NASA’s Dr Gavin Schmidt points out in a RealClimate blog post, it is still unclear why short-term forecasts should necessarily be linked to sensitivity. Schmidt suggests that “it may be that all models that do well on this task [short-term forecasts] have a range of ECS values, and that the coincidence of this one model doing well and having a high ECS, was just that, a coincidence”.
These new high-sensitivity models are a reminder that large uncertainties (and long tails of risk) remain, but climate models are just one of many lines of evidence that scientists use to determine the sensitivity of the climate. Given the range of new model sensitivity estimates, scientists suggest that it is still premature to say that climate sensitivity is likely higher than previously thought.
CMIP6 historical simulations
With CMIP6 still running behind schedule, only 35 different models of the eventual 100 or so have so far submitted future emissions scenario runs. While results may change as other modelling groups finish, there are enough models available to provide an assessment of how CMIP6 will likely turn out compared to CMIP5.
It appears increasingly likely that not all CMIP6 runs will be available by the deadline to be included in the final IPCC report, due to be published in April 2021. However, CMIP6 runs not ready in time for the AR6 will still be used by researchers in the future.
While climate models output comprises thousands of different climate variables, a lot of attention is focused on global surface temperatures. Two different experiments that examine surface temperatures are of particular interest: the model surface temperature “hindcast” – where modellers simulate the period from 1850 through to the present day – and the projections of future warming under different emission scenarios.
Hindcasts are a useful tool for modellers to assess the performance of climate models. When models do a good job of representing past changes, it can instill confidence that they will get future changes correct as well.
Climate models cannot resolve all the small scale physics of the Earth’s climate given the limitations of computing power available today. In some cases they have to provide values for processes that occur at too small a scale to effectively simulate – such as cloud formation. The choice of these values – which often are themselves highly uncertain – is known as model tuning. While most modellers avoid explicitly tuning their models to match past temperature changes, when large mismatches are discovered it may send them scrambling to find a fix. In CMIP6, a few modelling groups explicitly tune some parameters to better match historical temperatures – though most still do not.
The figure below shows the global surface temperature “hindcast” from climate models in both CMIP6 (blue) and CMIP5 (grey) compared to observations from the NASA GISTEMP dataset. Lines show the average of the 42 CMIP6 models currently available that provide hindcasts, while the shaded area shows the 95% confidence interval of model runs.
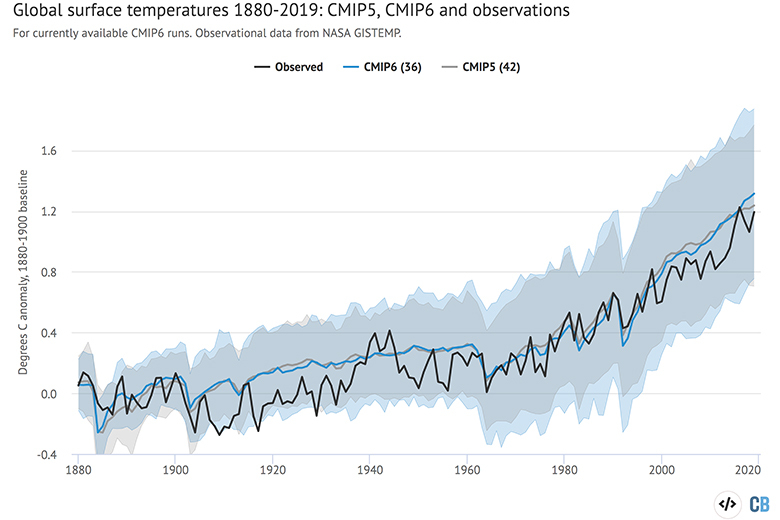
Despite the changes to ECS values in CMIP6, the hindcast is quite similar to that of CMIP5; observations are nearly always within the range of model runs and models capture modern warming reasonably well. CMIP6 models do not do a particularly good job simulating more-modest early 20th century warming observed from 1900-1940, though in this respect they are similar to CMIP5.
One way to evaluate hindcast performance is to compare the rate of warming in models and observations over a period of time. The figure below shows the rate of warming in observations (from NASA), CMIP5 models and CMIP6 models for both the full 1880-2019 period (left), and the more modern 1970-2019 period (right). This analysis starts in 1880 because the observational record used does not extend all the way back to 1850. The labelled dots represents the average of all the models (the “multi-model mean”), while the uncertainty (blue lines) reflects the range of warming across all the models (or the uncertainty in rate of warming of the observations).
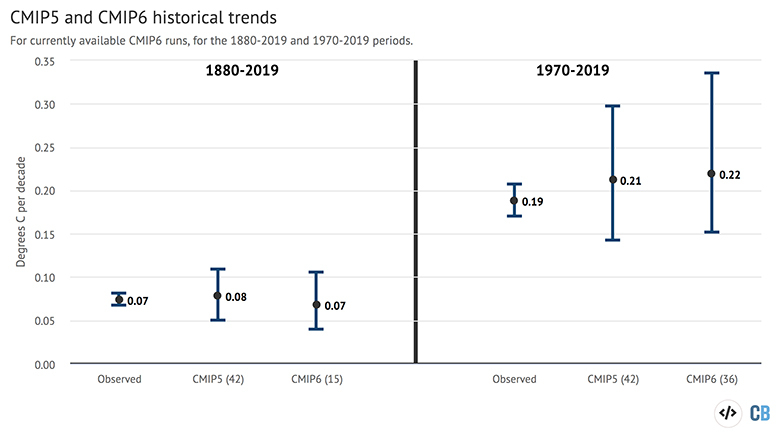
Over the full 1880-2019 period, both CMIP5 and CMIP6 match the observed rate of warming. Over the more recent 1970-2019 period, the multi-model mean in CMIP5 is warming 10% faster than observations, while the multi-model mean of the subset of CMIP6 models available so far is warming around 16% faster than observations. This higher warming rate in CMIP6 may be driven by the higher model sensitivity values.
Future warming in CMIP6
The limited number of future projections available in CMIP6 means that the collective results may change as more model runs come in. Nevertheless, an initial assessment of the level of future warming in the models available today is still possible. While the average of all the models will change as more results are available, it is clear that the range of values warming in CMIP6 will be notably wider than in CMIP5.
The figure below shows the range of warming and multi-model mean warming – from pre-industrial levels to 2090-2100 – for the four “tier 1” CMIP6 scenarios (SSP1-2.6, SSP2-4.5, SSP3-7.0, and SSP5-8.5) as well as the SSP1-1.9 scenario where a relatively large number of runs are available. The other scenarios are not shown in this figure, as there are still too few published runs to provide a comparable analysis. The numbers in brackets show how many modelling groups have currently submitted model runs for each scenario.
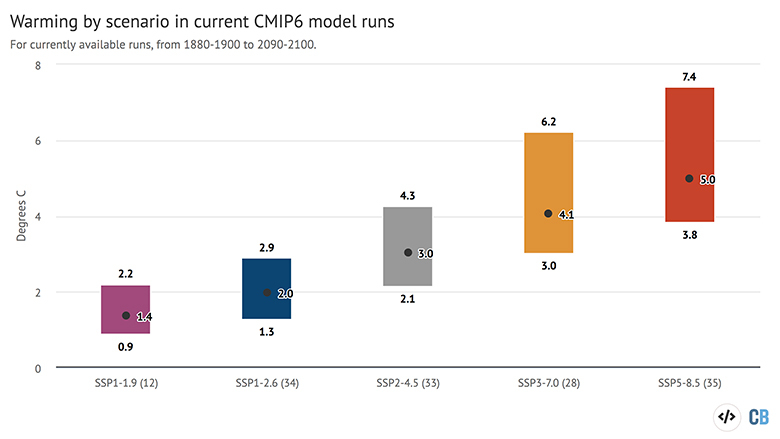
The results so far show large amounts of future warming; the new SSP1-1.9 scenario – intended to limit warming to 1.5C – has a multi-model mean warming of 1.4C. Similarly, the SSP1-2.6 scenario – which is analogous to the “well-below 2C” RCP2.6 of AR5 – shows mean warming of 2.0C. On the high end, the SSP5-8.5 scenario shows a mean warming of 5.0C, while the new SSP3-7.0 scenario shows 4.1C of warming. These values are modestly lower than those shown in the initial version of this article published in early 2020, as many of the more recently reporting models have slightly lower climate sensitivity – more in-line with values found in CMIP5 – than the early-reporting models.
Some future scenarios have relatively few runs available so far, so the initial values should be treated with caution (particularly for the multi-model mean) until more models finish their runs. Even those such as SSP2-4.5 – with 14 models reporting results – may change notably once the remaining models finish their runs and are added to the CMIP6 database.
The results so far differ fairly substantially from those found in CMIP5 for similar forcing scenarios. The figure below compares warming from pre-industrial levels between similar scenarios in CMIP5 and CMIP6.
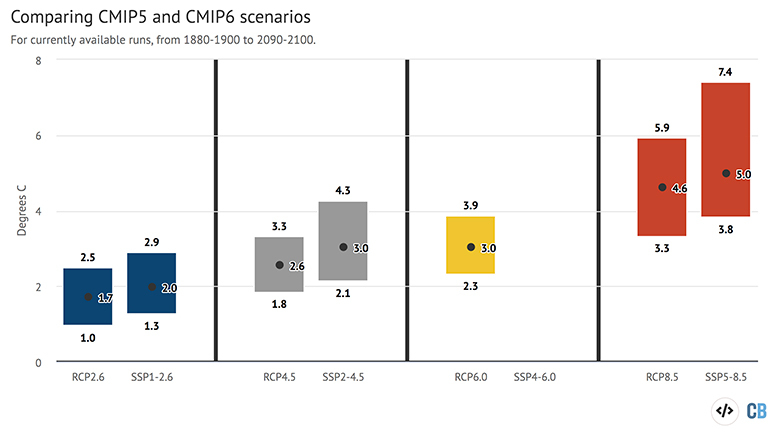
While RCP2.6 had a multi-model mean estimate of 1.7C warming in CMIP5, the new SSP1-2.6 scenario has an average warming of 2.0C. Similar differences are found for the other scenarios, with CMIP6 variants showing around 0.4C more warming. Note that CMIP6 results are not shown for SSP4-6.0, as it is a “tier 2” scenario with too few model runs currently available to produce an estimate comparable to the other scenarios. Differences between RCP and new SSP scenarios also have a small impact on end-of-century warming.
More models and experiments
Experiments in CMIP6 include basic “diagnostic” simulations (called DECK) where CO2 is increased by 1% per year, or suddenly quadrupled, or where the climate forcings are left relatively unchanged for long periods of time. These include historical runs driven by observed changes in CO2 and other climate forcings, and future emissions scenarios through the 21st century and beyond.
In addition, there are 22 specialised experiments (23 including future emissions scenarios) that modelling groups can choose to participate in. These experiments – called Model Intercomparison Projects, or MIPs – provide useful assessments of changes to the climate beyond those in the basic diagnostics and historical simulations. For example, in the GeoMIP experiment, different modelling groups simulate the effect of different types of geoengineering on the climate. The MIPs included in CMIP6 are shown in the figure below.
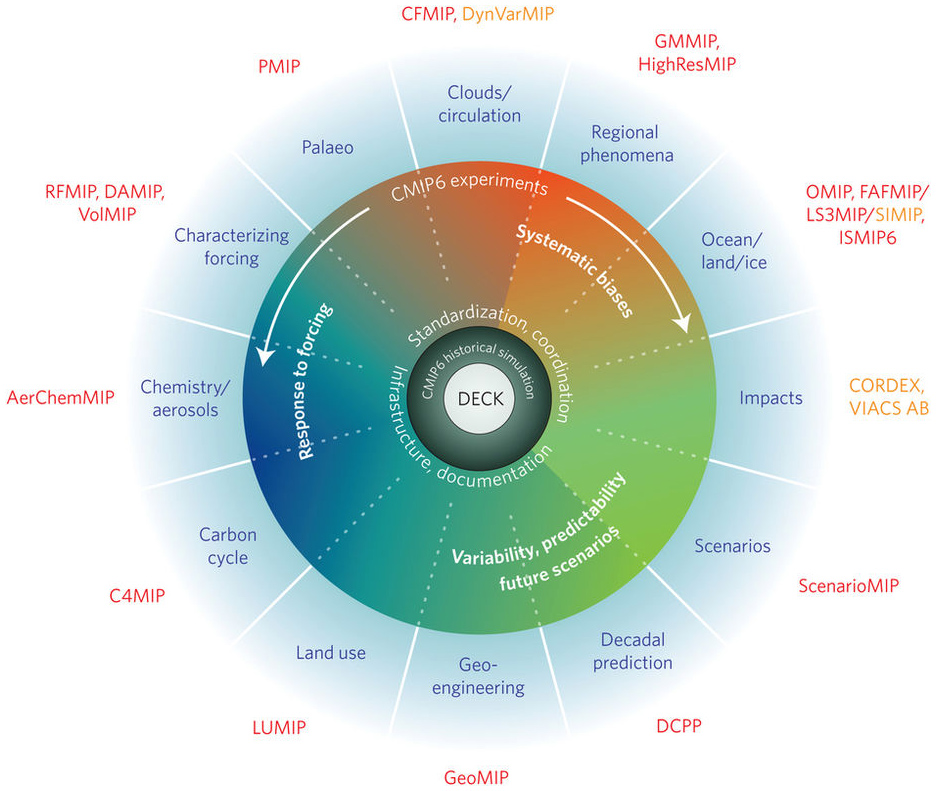
Not all modelling groups will participate in all MIP experiments in CMIP6 – in part because not all groups have the same level of supercomputing resources or interest in particular experiments. The MIPs included in CMIP6 are listed below; details about each can be found in the accompanying links.
- The Aerosols and Chemistry Model Intercomparison Project (AerChemMIP)
- Coupled Climate Carbon Cycle Model Intercomparison Project (C4MIP)
- The Carbon Dioxide Removal Model Intercomparison Project (CDRMIP)
- Cloud Feedback Model Intercomparison Project (CFMIP)
- Detection and Attribution Model Intercomparison Project (DAMIP)
- Decadal Climate Prediction Project (DCPP)
- Flux-Anomaly-Forced Model Intercomparison Project (FAFMIP)
- Geoengineering Model Intercomparison Project (GeoMIP)
- Global Monsoons Model Intercomparison Project (GMMIP)
- High-Resolution Model Intercomparison Project (HighResMIP)
- Ice Sheet Model Intercomparison Project for CMIP6 (ISMIP6)
- Land Surface, Snow and Soil Moisture (LS3MIP)
- Land-Use Model Intercomparison Project (LUMIP)
- Ocean Model Intercomparison Project (OMIP)
- Polar Amplification Model Intercomparison Project (PAMIP)
- Palaeoclimate Modelling Intercomparison Project (PMIP)
- Radiative Forcing Model Intercomparison Project (RFMIP)
- Scenario Model Intercomparison Project (ScenarioMIP)
- Volcanic Forcings Model Intercomparison Project (VolMIP)
- Coordinated Regional Climate Downscaling Experiment (CORDEX)
- Dynamics and Variability Model Intercomparison Project (DynVarMIP)
- Sea Ice Model Intercomparison Project (SIMIP)
- Vulnerability, Impacts, Adaptation and Climate Services Advisory Board (VIACS AB)
There are 49 different modelling groups participating in CMIP6, up from around two dozen in CMIP5. The map below shows the location of the modelling groups participating in CMIP6.
The output that each modelling centre produces for CMIP6 is loaded on a central web portal, managed by the Program for Climate Model Diagnosis and Intercomparison (PCMDI), which scientists across many disciplines and from all over the world can then freely and openly access. There is also a special issue of the journal Geoscientific Model Development on CMIP6, with 28 published papers covering the overall project and the specific MIPs.
CMIP6 is a huge modelling effort, substantially more ambitious than CMIP5. This has led to some delays, with CMIP6 currently running at least a year behind schedule. While the IPCC AR6 is currently being drafted, only a relatively limited set of models are available, and it seems unlikely that all CMIP6 runs will be completed in time for the final AR6 draft.
Acknowledgements
Dr Christine McKenna at the University of Leeds and the CONSTRAIN project made model runs available to create the figures in this analysis.