Received 29 September 2015; accepted 27 November 2015; published 30 November 2015


1. Introduction
Ginger (Zingiber officinale) (Family: Zingiberaceae) is an herbaceous perennial, the rhizomes of which are used as a spice. India is a leading producer of ginger in the world and during 2012-13 the country produced 7.45 lakh tonnes of the spice from an area of 157,839 hectares. Ginger is cultivated in most of the states in India [1] . However, states namely Karnataka, Orissa, Assam, Meghalaya, Arunachal Pradesh and Gujarat together contribute 65 percent to the country’s total production. Ginger originated in Asia and now grows in several parts of the world. The root of the plant is harvested for many culinary and medicinal uses. It can be used in many forms and is edible raw as well as cooked. One of the most common uses of ginger is to relieve nausea and other gastric ailments.
A flowering plant is actually the root of the ginger plant that is harvested. A single root can have many offshoots above ground. The portion of the plant above ground has slender stalks with long leaves that come to a point. The flowers grow in clusters and are green and purple. Ginger is a tropical plant that is found in the Caribbean, India, Southeast Asia, and West Africa. Though the full name is root ginger, it is usually just called ginger.
Traditionally ginger plant is harvested manually to get the ginger out of ground. But it consumes more time and more physical exertion of worker. It is necessary to introduce machine to harvest to reduce time consumption and human efforts. A machine of this nature can be fabricated at village level application in India such as to harvest at minimum time and a minimum cost, to get ginger as quickly as possible from the field, to reduce the physical exertion, to avoid physical damage to Ginger, to reduce man power and to ensure the safety to labors.
2. Processing of Ginger
Processing of ginger to produce dry ginger basically involves two stages-peeling of the ginger rhizomes to remove the outer skin and sun drying to a safe moisture level. Peeling―Peeling serves to remove the scaly epidermis and facilitate drying. Peeling of fully matured rhizomes is done by scrapping the outer skin with bamboo splits having pointed ends and this accelerates the drying process. Deep scraping with knifes should be avoided to prevent the damage of oil bearing cells which are present just below the outer skin. Excessive peeling will result in the reduction of essential oil content of the dried produce. The peeled rhizomes are washed before drying.
The dry ginger so obtained is valued for its aroma, flavour and pungency. Indian dried gingers are usually rough peeled when compared to Jamaican gingers, which are clean peeled. The rhizomes are peeled only on the flat sides and much of the skin in between the fingers remains intact. The dry ginger so produced is known as the rough peeled or unbleached ginger and bulk of the ginger produced in Kerala are of this quality.
3. Methodology
3.1. Force Determination
Before going to design a harvesting machine, it is necessary to know the force required to dig/penetrate the ginger bed and get ginger out of bed shown in Figure 1. The set up was fabricated as per the drawing shown in Figure 3 to determine the force required to penetrate the blade into the bed. The blades are similar to pick-axe in construction, and then fabrication was carried out .The legs i.e. structural support were placed along the bed in such way that the bed has to come exactly at middle of the legs. By conducting this experiment (by trial and error method), it was concluded that the force/load required to penetrate the blade into the bed is 42 kg (considering the maximum force). Figure 2 shows Bed and Trench Representation and Table 1 represents force reading taken in ginger fields.
![]()
Figure 1. Arrangement of force determination.
![]()
Figure 2. Bed and trench representation.

Selection of Engine
Since the 50 - 60 rotation per minute is required for the blades of machine to get ginger out of bed. Hence the power of the engine is given by (N);

Hence Auto front engine is selected which has capacity 150 cc.
3.2. CAD Model
Figure 3 shows assembly drawing of ginger machine done by CATIA V5. It contains various parts such as C- Channel, blades, engine, bearing, chain sprocket and blade cup.
3.3. Design of Chain Drives
Selected chain shown in Figure 4―L 85 SL [2] (from iwis designation)
Design parameters of chain drives
1) Pitch of the chain (Assuming the Pitch of the chain to be 12.7 which is commercially available in the market).
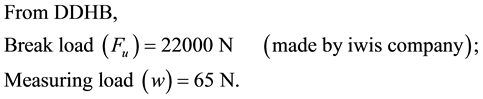
2) Number of teeth on sprockets
Assuming the number of teeth of small sprocket (driver sprocket) to be 11,
We have;
Velocity ratio (i) = number of teeth on driven sprocket/number of teeth on driver sprocket;

3) Pitch diameter of sprockets

4) Velocity of sprocket

5) Required pull

6) Allowable pull 

7) Number of strands

8) Check for actual factor of safety
![]()
9) Length of chain in pitch (Lp)
![]()
Centre distance
.
It implies that,
.
Therefore,
.
Selecting the nearest even no. of pitches is 76.
10) Length of chain
.
11) Correct centre distance (C)
![]()
3.4. Design of Shaft
Figure 5 shows design of shaft. In order to design a rotary tiller, the special work of the tiller and also the performable work of the tractor should be determined. The specific work of rotary tiller is defined as the work carried on by rotary tiller at each rotation of tillage blades per the volume of broken soil, which could be calculated by the following equation (Bernacki et al., 1972) [3] :
(1)
where: AO and AB are the static specific work and dynamic specific work of rotary tiller (Kg-m/dm3), respectively, which can be calculated throw the following equations (Bernacki et al., 1972):
(2)
(3)
(4)
where: Co is the coefficient relative to the soil type, Ko is the specific strength of soil (kg/dm3), u is the tangential speed of the blades (m/s), v is the forward speed (m/s), au and av are dynamical coefficients that are relative together throw the following equation:
(5)
where:
(6)
The performable work of the tractor (Ac) could be calculated by the following equation (Bernackiet al, 1972):
(7)
where: Nc is the power of tractor (hp), v is the forward speed (m/s), ç cis the traction efficiency that its value for the forward rotation of the rotary tiller shaft is 0.9; whilst the value for the reverse rotation of the rotary tiller is considered between 0.8 - 0.9, çz is the coefficient of reservation of tractor power which is between 0.7 - 0.8, a is the rotary tiller work depth (dm) and b is the tiller work width (dm). Matyashin (1968) reported that at the forward rotation of the rotary tiller shaft, the tillage power consumption is decreased 10% - 15%, in comparison with the shaft reverse rotation. Hence, in this design the forward rotation was considered for the rotary tiller shaft to reduce the tiller power consumption and also utilization of the rotary tiller thrust force at the forward rotation. In designing the rotary tiller, the hard condition of the soil was considered. The values of Co, Koandauin very heavy soils are 2.5, 50 (kg/dm3), and 400 (kg.s2/m4), respectively (Bernacki et al., 1972). Therefore, the static special work of the rotary tiller could be calculated by replacing the values in the Equation (2):
![]()
Since the values of b, v, ë and av are not given in the Equations (4), (5) and (7), a proper domain should be defined for the values at first, with respect to the technical specifications of the selected power tiller for this design. Then, the optimum condition for the rotary tiller design could be selected from the domain. The recommended work width for the power tiller model of Mitsubishi VST SHAKTI 130DI is 60 cm. The distance between rotary tiller flanges in this design was considered equal to 20 cm. Therefore, the work width domain of the rotary tiller that is the multiple of the distance between the flanges is in the range of 50, 60, 70 and 80 cm. This range was selected with respect to the power tiller work width. The forward speed of the power tiller model of Mitsubishi VST SHAKTI 130DI at different transmission gears are presented in Table 2. Because at the high levels of power tiller forward speeds, the penetration ability of the rotary tiller blades in the soil reduces, in this design only the heavy transmission gears were considered as the domain of forward speed. Hendrick and Gill (1971) suggested the minimum value of 2.5 for ë [4] .
Hence, a domain from 2 to 22 and from 0.2 to 2 was considered for ë and forward speed, respectively, to provide a large section range for the rotary tiller design. According to the explanations offered above and by Equations (1), (4) and (7), the values of A and Ac could be calculated:
(8)
(9)
By using Equations (8) and (9), the values of A and Ac with respect to the defined domain for ë and v are obtained in Table 3 (Mohammadi Alasti et al., 2008).
![]()
Table 2. The forward speed of the power tiller model of mitsubishi VST SHAKTI 130DI at different transmission gears.
![]()
Table 3. The values of specific work of rotary tiller and maximum work of power tiller at different values of V and Λ.
The proper selection of the forward speed is dependent to the tangential speed of the blades (that is a function of rotational speed of rotor) and the length of sliced soil. The tangential speed of the blades (u), the rotational speed of the rotor (n), and the length of sliced soil (L) could be obtained by the following equations:
(10)
(11)
(12)
In the equations, R is the rotor radius (cm), v is the forward speed (m/s) and Z is the number of blades on each side of the rotor flanges. In this design, two blades were considered on each side of the flanges (Z = 2). The working depth selected for the rotary tiller in this design was 15 cm .The conventional diameter for rotary tillers rotor is variable from 30 to 50 cm. Moreover, the radius of rotor for rotary tillers should be selected greater than the working depth (Matyashin, 1968).Considering these explanations, a 50 cm diameter was diagnosed to be appropriate for the rotary tiller rotor. By replacing the selected value for the rotor diameter in the Equations (11) and (12), we will have:
(13)
(14)
The total possible selections for the rotary tiller working width (b), forward speed (v) and rotational speed of rotor (n) are presented in Table 3. Table 3 is obtained through Equations (13) and (14). Firstly, for each of the selected working widths in Table 3, the closest value of the rotary tiller special work to the performable work of the power tiller was determined at each of the forward speeds. Then, the corresponding values of ë for each forward speed were determined to calculate the rotor speed and the length of sliced soil (Table 3). By selecting the rotary tiller special work and the performable work of the power tiller close together at each of the forward speeds, an appropriate conformity will be continued between the rotary tiller and power tiller.
Kepner (1972) reported that the power needed of the tractor PTO for supplying a rotary tiller should be approximately 1 hp for each centimeter of working width. Considering the suitable domain obtained for the rotor speed, the length of sliced soil and the forward speed, at the working width of 70 cm, this width was selected as a proper working width for the power tiller. Moreover, at the selected working width there was a little difference between the rotary tiller special work and the performable work of the power tiller (Table 4).
Considering the results presented in Table 4, it becomes evident that the selected power tiller for this design only at the gear one can supply a rotary tiller with the working width of 70 cm and working depth of 15 cm.
After specifying the appropriate working width for the power tiller, the length of sliced soil, the rotational
![]()
Table 4. Total possible selections for the rotary tiller working width, forward speed and rotational speed of rotor.
speed of the rotor and the tangential speed of the blades should be calculated at the selected gear (the forward speed of 0.50 m/s). Before performing the mentioned calculations, the appropriate value of ë proportional to the selected forward speed for the power tiller should be obtained. For this purpose, the special work of the rotary tiller and the performable work of the power tiller should be equal together [5] . Therefore,
By representing the obtained value for ë at the Equations (10), (11) and (12) we will have:
;
;
.
Design of Rotor Shaft
For designing the rotor shaft shown in Figure 5, the maximum tangential force which can be endured by the rotor should be considered. The maximum tangential force occurs at the minimum of blades tangential speed is calculated by the following (Bernacki et al., 1972):
(16)
Cs is the reliability factor that is equal to 1.5 for non-rocky soils and 2 for rocky soils (Berbacki et al., 1972). From Equations (6) and (12), it becomes evident that umin is obtained at λmin; and λmin is obtained at Lmax. So, we will have:
![]()
![]()
By representing the values of λmin and umin at the Equation (16), the maximum tangential force on the rotary tiller shaft will be obtained:
![]()
The maximum moment on the rotor shaft (Ms) is calculated through the following:
![]()
In the above equation, R is the rotor radius (cm).
Considering the results obtained above, the rotor should be made from roll steel (AISI 302) having yield stress of 520 MPa. The allowable stress on the rotor (τall) is calculated by the following equation (Mott, 1985):
(17)
where sy = 500 MPa.
In the equation, k is the coefficient of stress concentration equal to 0.75 and f is the coefficient of safety, which is equal to 2. By replacing the values of k and f in the Equation (17):
![]()
The torsional moment is the most important factor that significantly affects the rotor shaft design (Yatsuk et al., 1981). Considering the equation for calculating the torsional moment on rotating shafts, the proper diameter for the rotary tiller shaft could be obtained [5] .
![]()
3.5. Design of Bearing
Design and Calculation (shown in Figure 6)
Diameter of bearing (bore size) d = 30 mm
Stationary load (Fr) = (weight of shaft + weight of blade) ![]()
Considering the higher end ≈ 300 N
Working hours = 5 hours, 5 days/week for 2 years
On the basis of given information,
Select 30 BC 02 SKF from DDHB;
Basic capacity dynamic load (Cr) =14710 N
Required rated dynamic load ![]()
Fe → Equivalent load ![]()
where V → Rotation factor = 1 (if inner race is rotating)
= 1.2 (if outer race is rotating)
Ka → load application factor = 1.5 for light shock
Kt → temperature factor = 1 (assuming bearing working at normal temperature)
Substituting, Fe = 1 × 300 × 1.5 × 1 = 450 N
Ld → design life = 5 × 5 × 52 × 2 =2600 hr
Lr → rated life = 500 hr
nd → design speed = 100 rpm
nr → rated speed = 100/3 rpm
Substituting, Cr = 300 × [(2600/500) × (100/(100/3))]1/3 = 749.599 N
Bearing No. 6206 is suitable since whose rated dynamic capacity is greater than the required. Hence selected bearing is 30 BC 02 SKF and its specifications are [6] ,
Bearing No. = 6206
Bore size (d) = 30 mm
Outer diameter (D) = 62 mm
Width (B) = 16 mm
Fillet radius = 1.5 mm
3.6. Design of Blade
Design of blade (shown in Figure 7)
Force (F) = 42 kg = 412.02 N
Assuming 150% more force for the initial dig
i.e. F = 618.03 N @ 618 N
Considering a section of a-b,
Inner radius (ri) = 90 mm
Outer radius (ro) = 120 mm
Distance of centroidal axis from the inner fibre
![]()
Radius of centroidal axis ![]()
Radius of neutral axis
![]()
Distance of neutral axis from centroidal axis ![]()
Area of cross section at a?a ![]()
Distance of neutral axis to inner radius
Distance of neutral axis to outer radius ![]()
Applied force (F) = 618 N
Bending moment ![]()
Direct stress ![]()
Bending stress at inner fibre
![]()
Bending stress at outer fibre
![]()
Combined stress at inner fibre
Combined stress at inner fibre
![]()
Maximum shear stress
![]()
At section b-b,
C1 = 13.33 mm & C2 = 17.667 mm
Area = 300 mm2
Moment of inertia
![]()
Direct stress ![]()
Bending stress at inner fibre ![]()
Bending stress at outer fibre ![]()
Combined stress (scomb) = −117.06 + 2.06 = −115.54 N/mm2 (compressive)
Maximum shear stress at section
![]()
3.7. Design of Fastener
M12 bolts are used to fasten the bearings to chassis (shown in Figure 8).
When the joint is not fluid tight then, ![]()
Core diameter d1 = 11.188107 mm
Therefore core area ![]()
For pre-stressed bolt, initial force (Fi) = allowable stress × core area of bolt
16828.08 = σ × 98.31
Therefore, allowable stress ( ) = 171.17 N/mm2
3.8. Design of Chassis
Chassis (shown in Figure 9) consists of L-Angular (shown in Figure 10) and C-channels as structural members.
3.8.1. L-Angles
Steel Angle is used in industrial maintenance, agricultural implements, transportation equipment, etc.
Material of L-Angles used: Mild Steel
§ Also called as: HR steel angle, Mild steel angle, steel L;
§ Applications: Frame work, braces, supports, cross members, etc.;
§ Workability: Easy to Weld, Cut, Form, and Machine.
Steel Angle or L-Angle is a hot rolled, mild steel angle shape with inside radius corners that is ideal for all
structural applications, general fabrication and repairs. Table 5 shows specifications of L-Angle which is used in machine.
3.8.2. C-Channel
C-Channel as shown Figure 11 used in industrial constructions, agricultural implements, transportation equipment, bridge constructions, etc.
Some of the characteristics are as follows:
§ Applications: Frame work, braces, supports, cross members, etc.;
§ It is widely used in supporting horizontal load;
§ Workability: Easy to Weld, Cut, Form, and Machine.
3.9. Orthographic Views of the Machine
Figure 12 represents orthographic views of the ginger machine.
3.10. Isometric View of Machine
Figure 13 represents isometric view of the ginger machine.
1) Parts Pictures
![]()
Rotary Shaft Small Chain Sprocket Long Chain Sprocket
![]()
Blade with cup holder Rotary Shaft with chain sprocket
![]()
Bearing with Casing C-Channels Chassis
2) Fabrication of Different Parts of Machines
Figure 12 and Figure 13 show orthographic views and isometric view respectively done by CATIA V5. Chassis frame is the main base of the machine on which all components are mounted with wheels and engine. As per the design, marking has done on each C-channels and L-angular. As per the marking, they are cut by cutting machine and holes are drilled by using drilling machine for fixing the plumber blocks to hold shaft along with blades assembly on the chassis are used.
The base C-channels are to 1000 mm length with the aid of cutting machine, four shafts of 25 mm diameter and length of 55 mm are welded using welding machine at distance of 920 mm. Six vertical C-channels of 800 mm and 560 mm are cut and welded on base C-channel. Two C-channels of 900 mm length and welded horizontally to the frame to provide platform for the Plummer blocks. The engine platform is also carried out in the same manner as shown in Figure 14 and Figure 15.
The blades are forged to the required shape and size, and fastened to blade cup holder with the aid of M10 × 1.25 bolts and nut. This assembly is then welded to shaft, in flute angled manner (the angle between each blade is approximately 20˚ to 25˚) at a distance of 75 mm. Then the shaft is fixed in Plummer blocks on either side of shaft. The engine is mounted on platform which is made on the chassis for transmitting power to rotary shaft.
The ginger harvesting machine consists of three main parts, they are:
1) Power Transmission System: This section provides power to the machine it mainly includes Auto front engine and gear box.
2) Digger: Blades are also called as digger which gets the ginger out from bed.
3) Screener: Screener is nothing but the mesh welded on the blade continuously as shown in Figure 15.
4. Working
The engine is started by kicking; the power which is available at output shaft of engine is transferred to the rotary shaft through chain drive. The required speed of 20 - 25 rpm is gained by the keeping smaller sprocket having teeth of 11 and larger sprocket of teeth 44.
![]()
Figure 14. Fully assembled ginger harvesting machine.
In running, the blades hit the bed one after the other not at a time so it is easy to move forward. The screener separates the ginger from soil and provides same by blades without affecting ginger. Then ginger has to be collected manually.
5. Results and Discussion
Comparisons are made between manual and machine harvesting shown in Figure 16. At present day ginger is harvested manually with the aid of labours, they demand Rs 100 per 60 kg of ginger (particularly in Hassan). In an hector (1 hector = 2.4710 acre) of land approximately 4.72 tonnes (47,200 kg) are grown [1] , so the total cost for an hector is approximate to Rs. 78,666. But using this machine 60 kg of ginger can be recovered Rs. 40 which includes the cost of petrol and person who will handle machine. The wage comparison between manual and machine harvesting is as shown in pie Figure 17.
![]()
Figure 17. Comparison of number of hours required to harvest one acre.
The machine takes minimum time to harvest ginger when compare to manual harvesting. Comparing with manual harvesting 50% of harvesting time and 60% of labours are reduced (in manual ginger harvesting 20 - 30 labours are required). The cost of harvesting is reduced by 34% when compare to manual harvesting.
6. Conclusion
The performance of the harvester is evaluated in the field. The cost of the machine is about Rs. 26,000/- and if the farmer buys this machine, he or she can recover the invested money back by harvesting two and half acres (approx.). It is quite simple in design and fabrication, so it can be fabricated at village level. By adapting this machine, problems of the labour crises can be reduced, when compared to manual harvesting with only 18% of labours being required. It makes the process faster than manual harvesting and hence reduces most of the harvesting time and labours required to operate the machine; thereby, it reduces the labour cost. The machine can be used by a maximum number of farmers; definitely farmer can overcome the labour crisis problem. The productivity can also be increased. This study leaves a wide scope for future investigations. In future it can be automated through tractors with the aid of PTO (Power Take Off) shaft. Collector can be used for collecting the ginger.